A University of Central Florida research team with collaborators at Virginia Tech have published critical findings about the electrochemical synthesis of ammonia, advancing sustainable fertilizer research and thus aiding global food safety efforts.
Ammonia, a compound of nitrogen and hydrogen, is an essential ingredient in many fertilizers for food production. However, its primary method of production, the Haber-Bosch method, is energy and fuel-intensive, consuming 3% to 5% of the world’s natural gas output and accounting for more than 1% of global carbon emissions.
Using the metal ruthenium as a catalyst, researchers identified the most efficient way to produce ammonia through a more sustainable production method — electrochemically. This production method can be more sustainable when electricity from renewable sources, such as solar or wind, is used to power the electrochemical synthesis, the researchers say.
The findings were published recently in ACS Energy Letters.
While there are many research efforts on electrochemical ammonia production, the underlying mechanisms have yet to be better understood, the researchers say.
However, this new research helps provide a clearer picture of the reaction mechanism, says study co-author Xiaofeng Feng, a professor in UCF’s Department of Physics
“The results of this in-depth work can provide important guidance to researchers on how to design more efficient catalysts towards sustainable ammonia production,” Feng says.
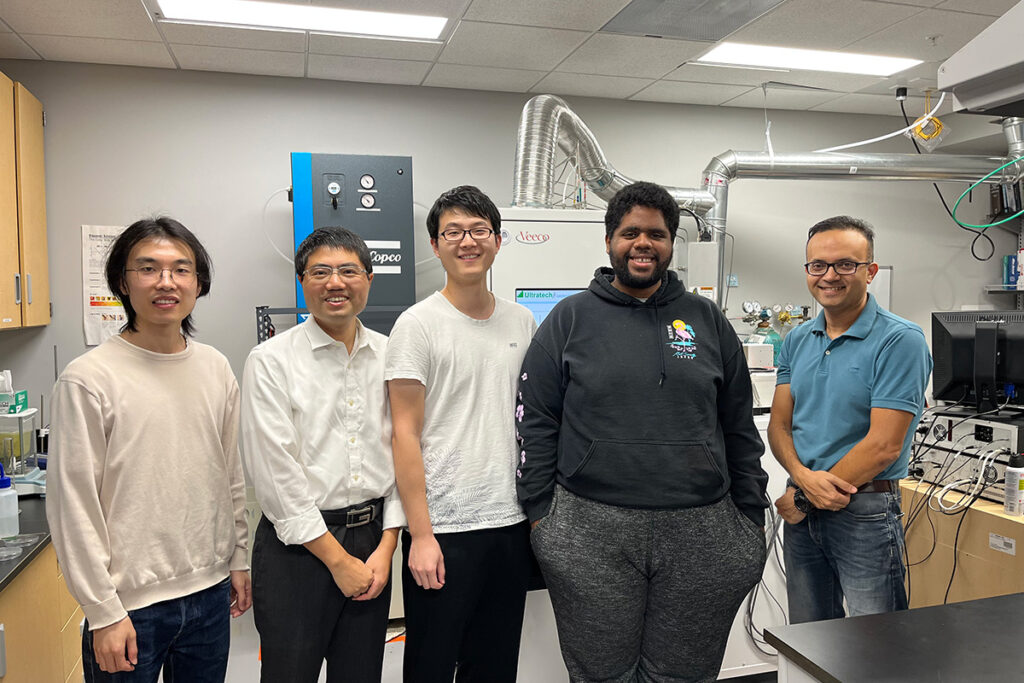
How They Did the Work
Ruthenium’s optimal binding strength with reaction intermediates makes it one of the most active catalysts for the nitrogen reduction reaction, which produces ammonia by combining nitrogen with hydrogen from water molecules.
Using atomic layer deposition, the researchers were able to have very precise control of the synthesized nanomaterials at the atomic scale, allowing the testing of ruthenium nanoparticles ranging from two to eight nanometers.
Researchers discovered that while layering ruthenium atoms into a catalytic structure, a special arrangement of ruthenium surface atoms — named the D5 step site — was the most active site for the electrochemical nitrogen reduction reaction.
Unlike other sites, the D5 step site possesses the “perfect balance,” favoring the formation of the N2H intermediate and not getting poisoned, or rendered unable to allow new molecules to adsorb and react, by the NH2 intermediate, the researchers say.
Ruthenium nanoparticles of around four nanometers in size were thus found to have the best catalytic performance for the nitrogen reduction reaction. Activity peaked at four nanometers and then dropped by five-fold as the particle size was doubled, demonstrating the critical effect of ruthenium particle size on the catalysis.
The researchers’ previous work to improve the efficiency of the electrochemical production of ammonia helped the current study by providing the mechanistic understanding and research methodology.
Collaborative Research
The new research is a collaboration between three research teams.
Feng and his students characterized the ruthenium samples and investigated them as catalysts for the electrochemical production of ammonia. Study co-author Parag Banerjee, a professor in UCF’s Department of Materials Science & Engineering, and his students focused on the precise synthesis of ruthenium metal nanoparticles in Banerjee’s lab.
Additionally, Virginia Tech professor Hongliang Xin and his student performed computational studies to model and identify the atomic structure that is responsible for the highest catalytic performance.
The researchers plan to collaborate further to develop more complex, efficient materials using atomic layer deposition for sustainable ammonia production, Feng says.
They also will implement the catalyst materials in advanced electrolyzer devices to improve the yield rate and efficiency of electrically powered ammonia production.
Researcher Credentials
Feng received his doctorate in materials science and engineering from the University of California, Berkeley in 2013 and joined UCF in 2016 as an assistant professor in the Department of Physics. The research in his lab is supported by an U.S. National Science Foundation CAREER Award grant.
Banerjee received his doctorate in materials science and engineering from the University of Maryland in 2011 and joined UCF in 2018.
The work in Banerjee’s lab was partially supported by the U.S. National Science Foundation and EMD Performance Materials.Banerjee and Feng are both members of the Renewable Energy and Chemical Transformations (REACT) Cluster, which facilitated the collaboration and supports more future opportunities.